Efficacy and Safety of Repetitive Transcranial Magnetic Stimulation in Postherpetic Neuralgia: A Systematic Review and Meta-Analysis
Article information
Abstract
This study evaluated the efficacy and safety of repetitive transcranial magnetic stimulation (rTMS) for pain management in postherpetic neuralgia (PHN). A comprehensive literature search was conducted through May 2024 in Scopus, PubMed, Web of Science, and Cochrane Library. Eligible studies included clinical trials, observational, and case-control studies. Two reviewers independently screened studies and extracted data. Risk of bias was assessed using RoB 2 for randomized controlled trials and the Newcastle-Ottawa Scale for observational studies. Meta-analysis was performed using Review Manager v.5.3, with heterogeneity evaluated by chi-square and I² tests. Five studies (245 patients) were included, with rTMS sessions ranging from 10 to 28. Meta-analysis showed significant pain reduction with rTMS compared to sham treatment. At 2 weeks post-treatment, the mean pain score difference (visual analogue scale) was –1.44 (95% confidence interval: –2.12 to –0.77; p<0.0001), with sustained relief at 1 and 3 months. However, no significant differences were found in the patient’s global impression of change scale, sleep quality, quality of life (QoL), medication regulation, or adverse events. rTMS exerted a consistent pain relief effect of rTMS, but its impact on broader aspects of patient well-being was less clear. rTMS provides sustained pain relief in PHN for up to 3 months, but its impact on QoL and secondary outcomes remains unclear, warranting further investigation.
INTRODUCTION
Postherpetic neuralgia (PHN) is a chronic neuropathic pain that occasionally follows an acute episode of herpes zoster infection.1 It is characterized by constant and often episodic sharp, burning, or stabbing pain and is commonly associated with a prolonged and potentially irreversible injury to the peripheral nervous system.1,2 The incidence of herpes zoster ranges from 1.2 to 3.4 per 1,000 persons per year among young individuals while incidence is 3.9 to 11.8 per 1,000 persons annually among individuals over 65 years.3 PHN is the most prevalent complication of zoster. Approximately 12.5% of zoster patients over the age of 50 have PHN, and the likelihood of such involvement increases with age.4 Such symptoms may have a wide range of effects on patients’ quality of life (QoL), impacting their physical and emotional well-being as well as their ability to carry on with normal daily activities.5
Conventional PHN management includes pharmacological treatments such as tricyclic antidepressants (TCA), alpha-2 delta ligands, anticonvulsants, topical analgesics, and opioids. However, frequently used oral medications have significant side effects that limit their practical use, especially with long-term drug use.6 Therefore, non-invasive therapies capable of relieving neuropathic pain without causing severe side effects are highly preferred.
Repetitive transcranial magnetic stimulation (rTMS) is a non-invasive treatment that employs magnetic pulses into the brain through the skull. rTMS directed at the primary motor cortex (M1) has been reported to help ease the pain of patients with neuropathic pain.7 Evidence demonstrates that rTMS administered at 5 Hz and 10 Hz frequencies is effective in improving pain relief, quality of sleep, and reducing anxiety in PHN patients.7 Applicability of rTMS to M1 has also been shown to have therapeutic benefit in various chronic pain conditions, such as complex regional pain syndrome and fibromyalgia.8,9
This study aims to assess the existing evidence regarding the effectiveness of rTMS in treating PHN. We aim to evaluate pain reduction, functional improvements, and safety issues with rTMS treatments in patients with PHN.
METHODS
We followed the Preferred Reporting Items for Systematic Reviews and Meta-Analyses (PRISMA) statement guidelines when reporting this systematic review and meta-analysis.10 All steps were done according to the Cochrane Handbook of Systematic Reviews and Meta-analysis of Interventions (ver. 5.1.0).11
1. Eligibility criteria
We included studies in our review if they satisfied the following criteria:
1) Population: patients with PHN
2) Intervention: rTMS
3) Comparator: sham or any other modality
4) Outcomes: (i) Primary outcomes: visual analogue scale (VAS) and patient’s global impression of change (PGIC) scales and (ii) Secondary outcomes: QoL, adverse events, medication regulations, sleep quality
5) Study design: clinical trials, observational cohort, case-control. We excluded review articles.
2. Search strategy
We searched the following electronic medical databases: Scopus, PubMed, Web of Science, and Cochrane Library from inception till May 18, 2024, using the following research query: (rTMS OR “Repetitive Transcranial Magnetic Stimulation” OR “Magnetic Repetitive Stimulation” OR “Transcranial Magnetic Stimulation Repetitive” OR “Transcranial magnetic stimulation” OR TMS) AND (“postherpetic neur*” OR “post-herpetic neur*” OR “Postherpetic polyneuropathy” OR “Post-herpetic polyneuropathy” OR post-herp* OR postherp* OR PHN OR “Herpes zoster neur*” OR “Herpes zoster pain” OR “Herpes zoster-associated pain” OR “Shingles pain” OR “Varicella zoster neur*” OR “Varicella zoster-associated pain”).
3. Screening and data extraction
The retrieved records were inserted into EndNote X9 to remove the duplicates and then added to the Rayyan database.12 Two authors (MEM and MAZ) blindly screened the articles based on title and abstract followed by full-text screening. In case of conflict, the first author (AA) resolved it. An Excel spreadsheet was used to extract the following data from the eligible studies: summary of the included studies (study design, duration, country, sample size, rTMS frequency, number of rTMS sessions, details of session, target location, outcome measured, and main findings), baseline characteristics of the included population (age, sex, pain duration, current medication, painful region, and the underlying disease), and the outcome measures.
4. Risk of bias assessment
Two authors (HS and MSMS) independently assessed the quality of the included studies. Randomized controlled trials (RCTs) were assessed using the Risk of Bias 2 (RoB 2) tool.13 The following domains were evaluated individually and graded as “low risk,” “high risk,” or “some concerns”: randomization processes, deviations from intended intervention, missing outcome data, measuring the outcomes, and selection of reported results. Case-control studies were assessed using the Newcastle-Ottawa Scale (NOS).14
5. Data analysis and synthesis
Statistical analyses were conducted using Review Manager v.5.3. We estimated the mean difference (MD) or standardized mean difference (SMD) in case of different scales in an outcome and 95% confidence intervals (CI) for continuous outcomes and the risk difference and 95% CI for binary outcomes. A p-value ≤0.05 was considered statistically significant.
We used the chi-square test to evaluate heterogeneity among the studies. If heterogeneity was found, the random-effect model was applied. All the statistical conversions were conducted by Meta-Analysis Accelerator software.15 Then, the chi-square statistic was used to calculate I2. A chi-square with a p-value less than 0.1 was considered significant heterogeneity. Also, the I2 value of more than or equal to 50% indicated high heterogeneity.7
Subgroup analysis according to follow-up time was performed. We performed sensitivity analyses (leave-one-out analysis) for significant results to determine the robustness of the effect size by removing one study per time to check the strength of the evidence and ensure the overall results were not altered. Since the included studies were lower than 10, we couldn’t conduct a publication bias.16
RESULTS
1. Search results and study selection
Database search yielded 68 citations, with 20 duplicates identified and removed. The remaining 48 studies underwent further evaluation. After reviewing titles and abstracts, 36 ineligible studies were excluded, leaving 12 potentially eligible studies for inclusion. All 12 full texts were retrieved and were thoroughly assessed by reading the full texts, and seven articles were subsequently excluded (Figure 1). In the end, five studies met the eligibility criteria for the systematic review, encompassing 245 patients.8-12 Out of the five studies, only four17-20 were included in the meta-analysis. The study by Wu and Liu21 could not be included in the meta-analysis because the control group received nerve block and pregabalin rather than sham treatment.
2. Characteristics of individual studies
The included studies’ summary, baseline, and outcomes are summarized in Table 1 and 2. There were four RCTs17-20 and one retrospective study,21 all conducted in China. The duration of the studies varied, ranging from 10 days to 36 weeks. The frequency of rTMS used was 10 Hz. In all studies, the target location was the M1, contralateral to the painful region.
3. Risk of bias and quality assessment
Regarding the RCTs, while Pei et al.18 and Wang et al.19 had an overall low risk of bias, the studies by Ma et al.17 and Chen et al.20 showed high risk of bias arising from the outcome measurement (Supplementary Table 1, available online). They also demonstrated some concerns regarding the randomization process. For the retrospective study, Wu and Liu21 showed fair quality according to the NOS score (Supplementary Table 2, available online).
4. Primary outcomes: visual analogue scale and patient’s global impression of change
The analysis of the VAS involved four studies17-20 comparing 10 Hz rTMS with sham, with 92 patients in each group. We found that 10 Hz rTMS showed significant improvement over the sham group after 2 weeks (MD: –1.44, 95% CI: –2.12 to –0.77, p<0.0001), 1 month (MD: –1.27, 95% CI: –1.99 to –0.56, p=0.0004), and 3 months (MD: –1.06, 95% CI: –1.75 to –0.38, p=0.002). The results were homogenous after 2 weeks (I2=0%, p=0.60) and 1 month (I2=47%, p=0.13) and heterogeneous after 3 months (I2=70%, p=0.02) (Figure 2).
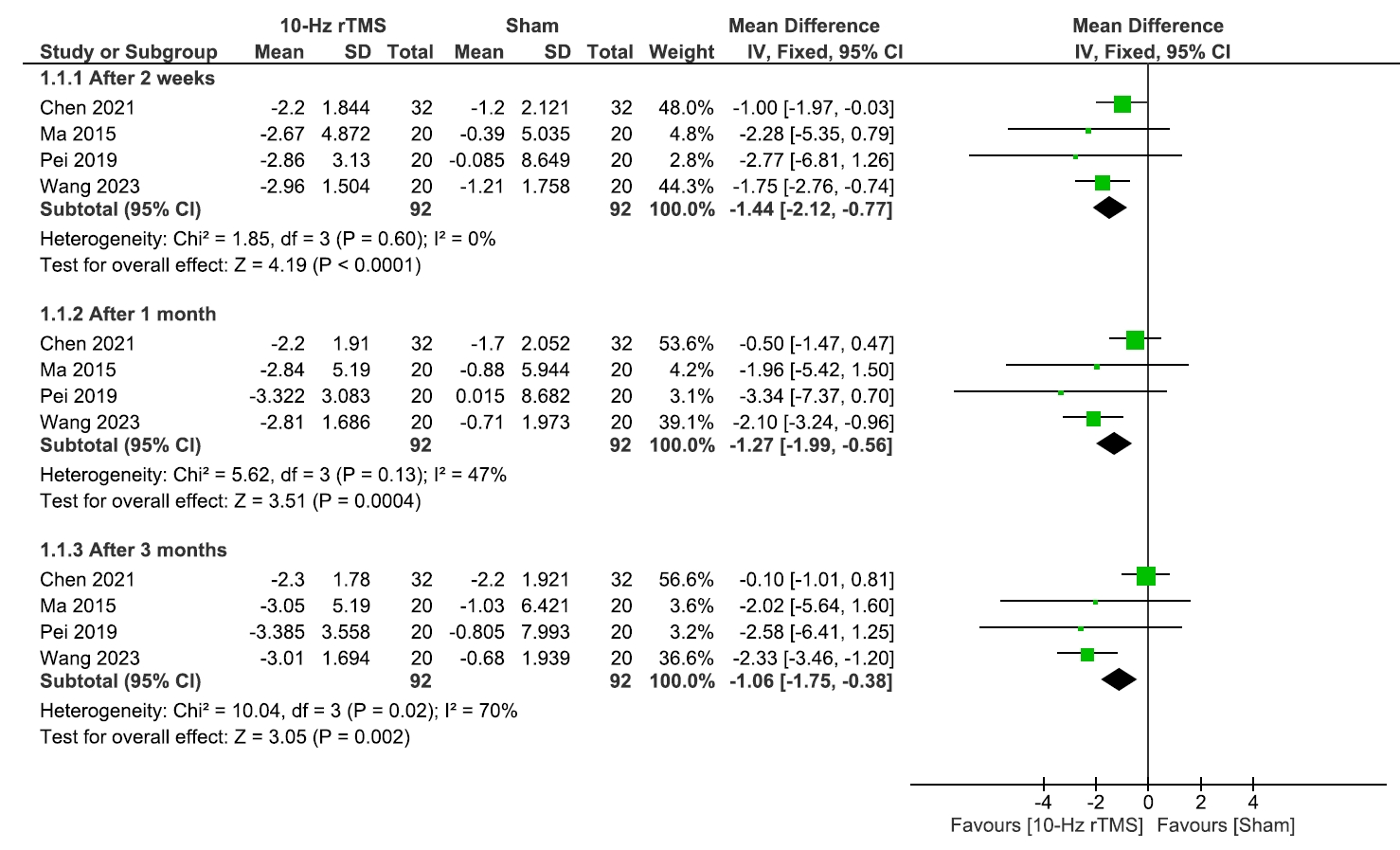
Visual analogue scale scale.
rTMS, repetitive transcranial magnetic stimulation; SD, standard deviation; IV, inverse variance; CI, confidence interval; df, degree or freedom.
Since the data was heterogeneous after 3 months, we conducted a leave-one-out analysis within the 3-month subgroup and found Chen et al.20 was the source of heterogeneity (I2=0%, p=0.98). Even after excluding it, our findings remained significant (MD: –2.32, 95% CI: –3.36 to –1.29, p<0.0001) (Supplementary Figure 1, available online).
Regarding PGIC which involved two studies17,18 with 40 patients in each group, no significant differences were observed between the 10 Hz rTMS and sham group after 10 days (p=0.36), 1 month (p=0.20), or 3 months (p=0.15). Results were homogenous (I2=0% for all, and p=0.89, p=0.61, and p=0.52, respectively) (Figure 3).
5. Secondary outcomes
1) Sleep quality
The analysis of the sleep quality involved three studies17-19 comparing 10 Hz rTMS with sham, with 60 patients in each group. We found insignificant differences between the two groups after 10 days (p=0.24), 1 month (p=0.19), and 3 months (p=0.20) (Figure 4).
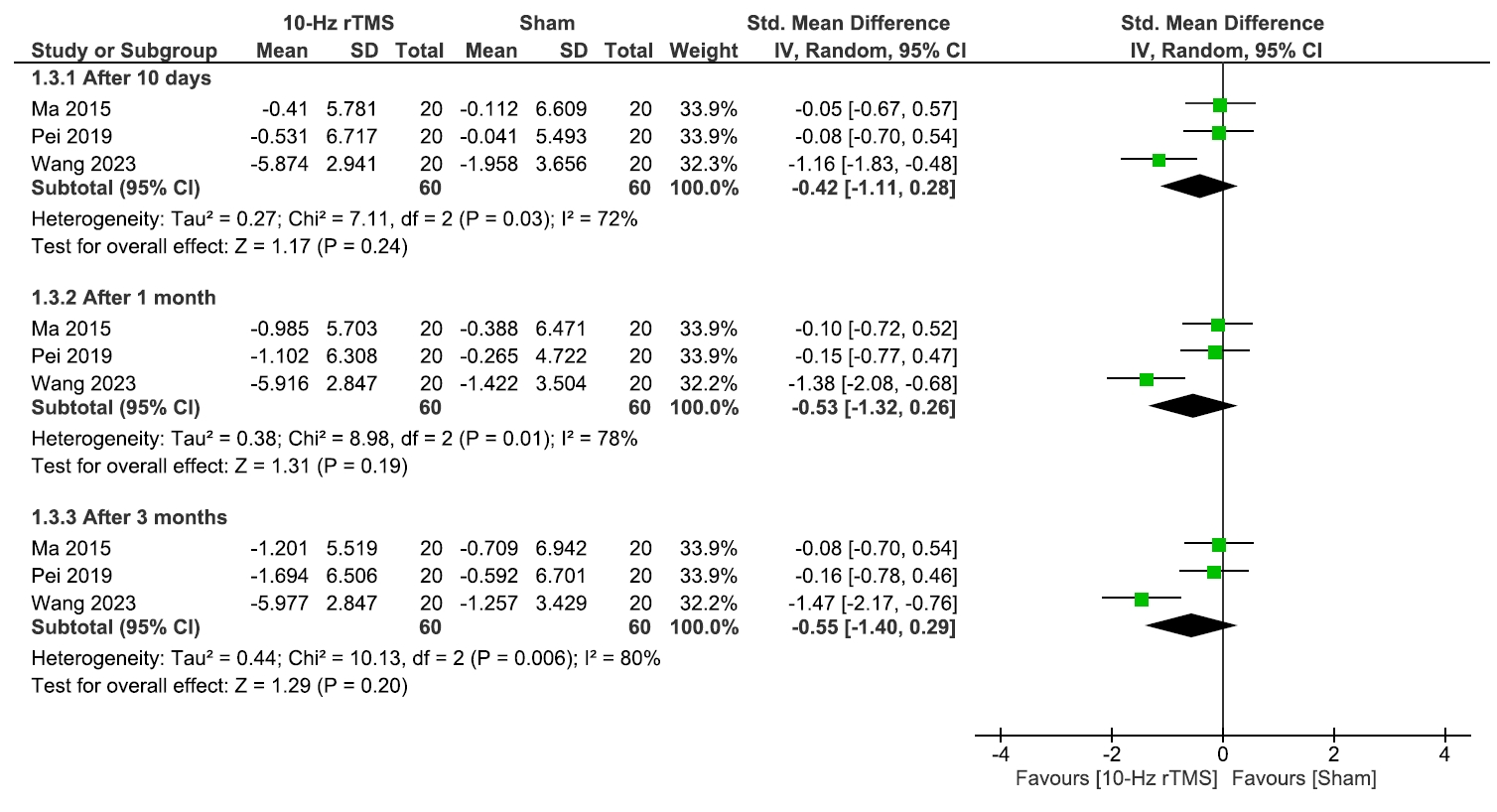
Sleep quality.
rTMS, repetitive transcranial magnetic stimulation; SD, standard deviation; IV, inverse variance; CI, confidence interval; Std., standardized; df, degree or freedom.
There was a statistically significant heterogeneity during these three periods, so we conducted a leave-one-out analysis which revealed that Wang et al.19 was the source of heterogeneity. However, the results remained insignificant after excluding this study (Supplementary Figure 2, available online).
2) Quality of life and medication regulation
No significant differences were observed between 10 Hz rTMS and the sham group regarding QoL (Figure 5), or medication regulation (Figure 6) after 10 days, 1 month, or 3 months.
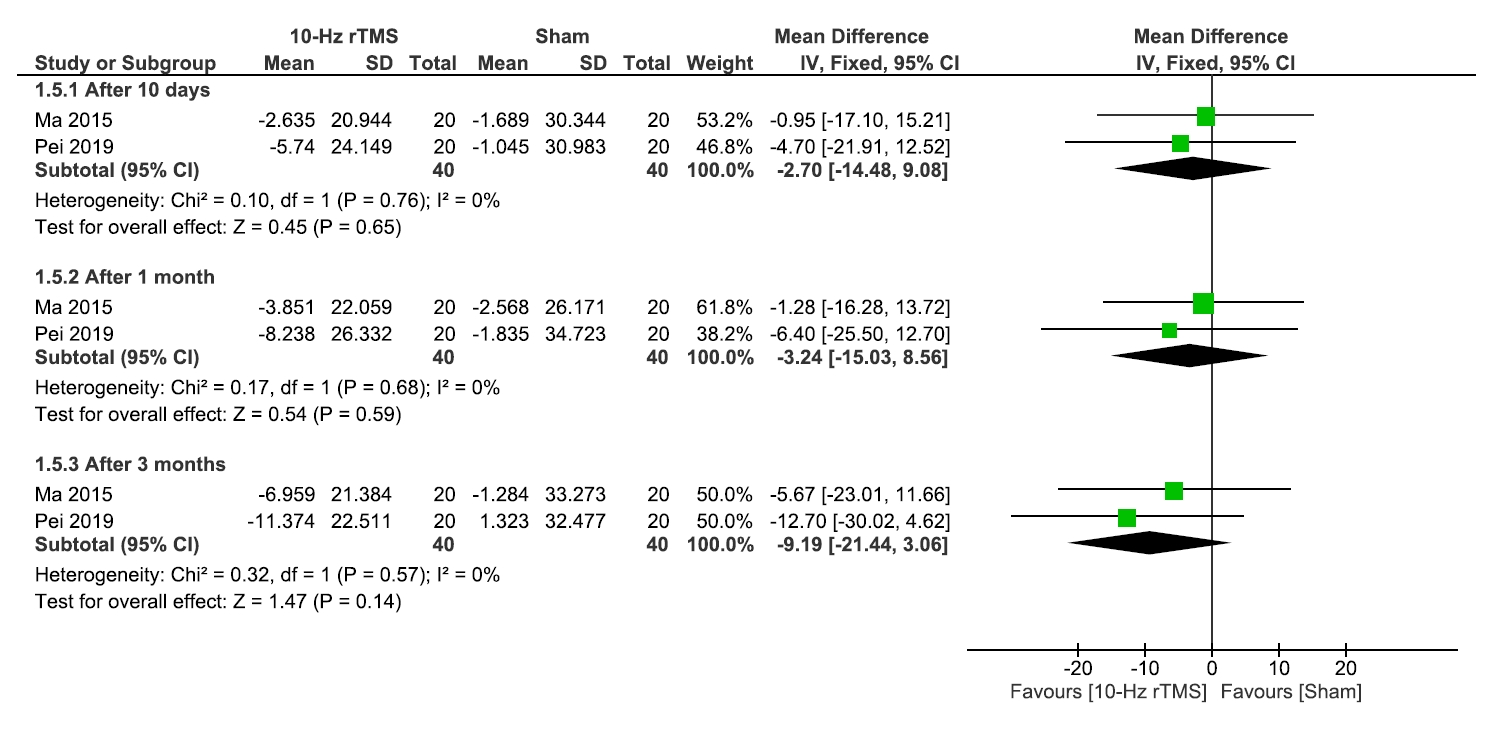
Quality of life.
rTMS, repetitive transcranial magnetic stimulation; SD, standard deviation; IV, inverse variance; CI, confidence interval; df, degree or freedom.
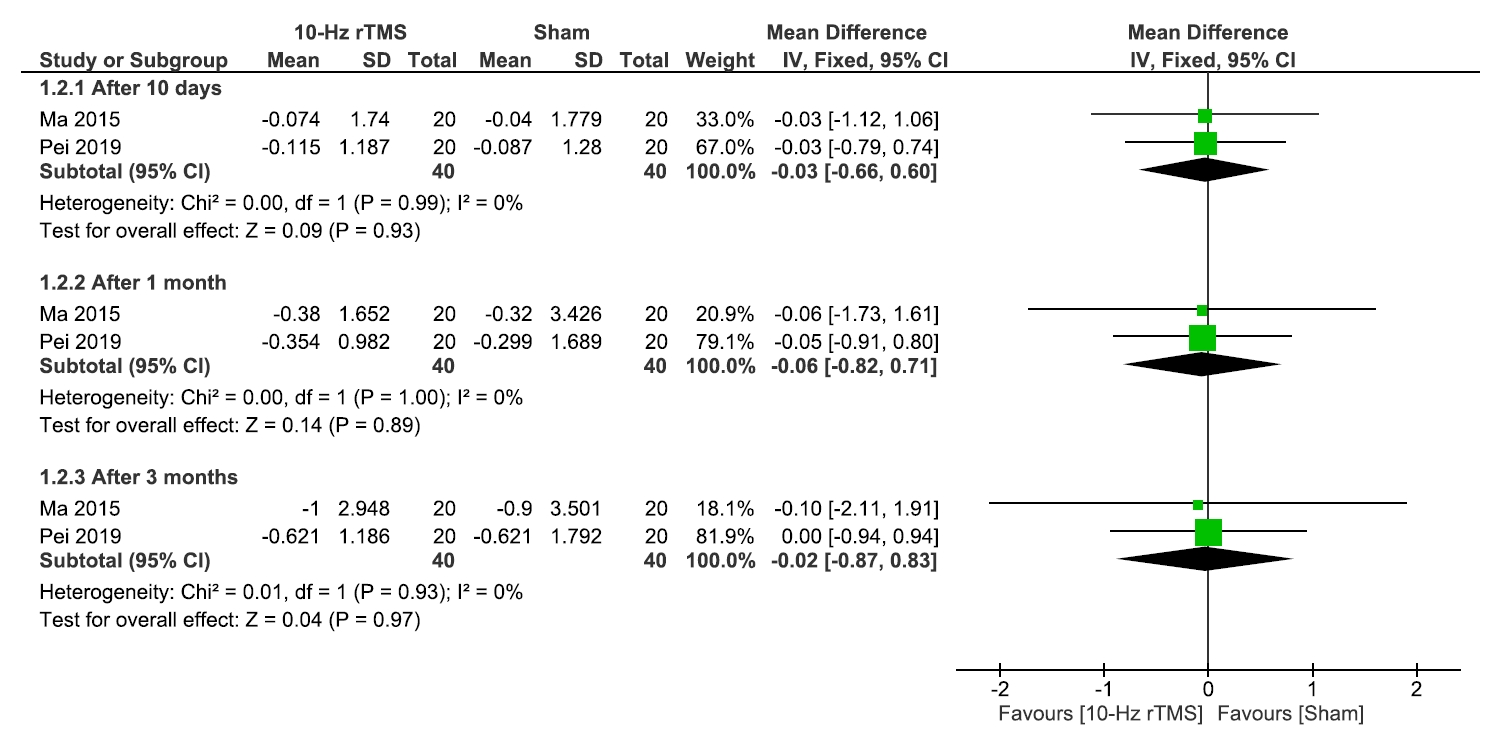
Medication regulation.
rTMS, repetitive transcranial magnetic stimulation; SD, standard deviation; IV, inverse variance; CI, confidence interval; df, degree or freedom.
Regarding the heterogeneity after 10 days, 1 month, or 3 months, results were homogenous for QoL (I2=0% for all, and p=0.75, p=0.68, and p=0.57, respectively) and medication regulations (I2=0% for all, and p=0.99, p=0.99, and p=0.93, respectively) (Figure 5, 6).
3) Adverse events
No significant differences were observed between 10 Hz rTMS and the sham group regarding the incidence of headache (p=0.77), dizziness (p=0.79), dry mouth (p=0.30), or neck pain (p=0.50), and results were homogenous (I2=0% for all, and p=0.54, p=0.95, p=0.99, and p=0.99, respectively) (Figure 7).
DISCUSSION
This study seeks to assess the efficacy of rTMS on M1 in PHN patients. We employed VAS pain scores and the PGIC scale as primary outcomes. Our meta-analysis found that rTMS significantly improved VAS pain scores compared to the sham group after 2 weeks (p<0.0001), 1 month (p=0.0004), and 3 months (p=0.002) across all studies, indicating an effective and long-lasting pain-relieving effect.
However, our study revealed no significant differences in PGIC score between the rTMS and sham groups at 10 days, 1 month, or 3 months of follow-up.
In our review, we included a total of five studies, four of which were RCTs and one was a cohort study. These studies encompassed patients who developed PHN following a herpes zoster infection, involving 245 patients. rTMS is notable for its swift pain-relieving effects, non-invasive nature, and minimal side effects, making it a significant advancement in the PHN pain management protocol.1-3 While the mechanism by which rTMs reduce pain is not very well understood, research indicates that its impact on the integrity of the corticospinal tract and thalamocortical tract is crucial for its pain-relieving effects.22 It also regulates the local cerebral blood flow and glucose metabolism, as well as the release of beta-endorphin, which acts as a pain mediator in the brain, while simultaneously inhibiting the release of nitric oxide synthase and reducing astrocyte activity.23-25 Interestingly, Moisset et al.26 suggested that rTMS also activates brain regions distant from the stimulation site in a mechanism similar to long-term potentiation, influencing glutamatergic networks and restoring cortical excitability, particularly endogenous opioids.
The primary target for rTMS was M1 contralateral to the painful side or corresponding to the dominant hemisphere. In some studies, the dorsolateral prefrontal cortex (DLPFC) served as an alternative target, alongside the M1 for the management of neuropathic pain.27,28 DLPFC stimulation for pain relief results in decreased activity in the thalamus, midbrain, and medulla.29 Other research indicates that the DLPFC can also impact neural regions involved in the emotional components of pain, such as the insular cortex and the anterior cingulate cortex.30 However, it is worth noting that results from two recent clinical trials show that stimulating the M1 rTMS region produces a more prominent analgesic effect compared to DLPFC rTMS stimulation.19,31
In our analysis, the pain-relieving effect of rTMS persisted for up to 3 months following treatment (Figure 2). The duration of pain relief from rTMS varies across studies. Some studies state that sustained effects as long as 3 months19 can be achieved while other studies report notable relief which is however short-lived.21 This variation indicates that there is a gap in knowledge on the factors that contribute to the maintenance of long-term pain relief.
All studies included in the analysis used 10 Hz rTMS for the therapeutic intervention. Pei et al.18 found possible efficacy in both 10 Hz and 5 Hz and proposed 5 Hz for first-line treatment for its safety, lower rates of side effects, and higher acceptability. However, both in that study and in our analysis, 10 Hz rTMS has demonstrated significantly superior effectiveness in achieving therapeutic benefits. Differences in rTMS parameters used across different studies, such as the frequency of stimulation, number of stimuli, interval between trains, and duration of sessions, contribute to the observed variability in the outcomes in the included studies and make it more challenging to interpret our findings.
First-line pharmacological treatments for PHN are oral TCA, pregabalin, and the lidocaine 5% patch.24 TCAs have a wide range of anticholinergic, antihistaminergic, and alpha receptor-blocking side effects that must be considered since the elderly and patients with reduced renal function are more susceptible to them.32 Despite opioids such as morphine and methadone having a great pain-relieving effect, their use in treating PHN is still controversial due to the risk of misuse, abuse, and addiction.33 Capsaicin patch and cream are also available alternatives, but not as well-studied as the lidocaine patch, and typically provide a lower level of pain relief overall than the lidocaine 5% patch.34 Other treatments include non-TCA and N-Methyl-D-aspartate antagonists; however, their effectiveness is supported by limited evidence and a concerning safety profile.35
Invasive treatment modalities include spinal cord stimulation (SCS), transcutaneous electrical nerve stimulation (TENS), behavioral therapy blocks (epidural, intercostal nerve, and stellate ganglion), and botulinum toxin injections.1,36,37
SCS is considered one of the most promising and innovative treatments.38 In a previous review of case reports on SCS, data from 11 reports were analyzed, and pain score data were available for 66 patients, showing an average pain relief of 79.0%. The average follow-up period was 50.8 months, suggesting a long-term relief effect.29 However, there is currently insufficient evidence regarding its efficacy in treating PHN, as most of the research conducted on its use consists of case reports.
Clinical trials have studied the combined use of TENS with other drugs commonly used in the treatment of PHN, such as pregabalin39 and antiretroviral drugs.40 Barbarisi et al.39 suggest that pregabalin produces superior outcomes when combined with TENS therapy, as indicated by a VAS score with p <0.02.32 Stepanović et al.40 investigated the use of TENS, antiviral agents, and a combination of TENS with antiviral agents for treating PHN following a herpes zoster infection. They found that the odds of developing subacute herpetic neuralgia were significantly the lowest in the group treated with TENS (odds ratio=0.15, 95% CI: 0.05–0.47, p=0.001).40
Pulsed radiofrequency therapy targeting the stellate ganglion and trigeminal ganglion shows promising results based on recent studies, as it reduces overall VAS scores and improves QoL. However, these studies were single-center designs with relatively small sample sizes.41,42 Botox injections are easy to administer and have few side effects; however, additional studies are needed to evaluate their efficacy.43
There has yet to be a definitive answer regarding the efficacy of rTMS with respect to other treatment principles, including deep brain stimulation or pharmacological medications. To determine the precise benefits and drawbacks of different therapy alternatives, comparative research needs to be carried out. Combining various treatments, such as medication or TENS, with rTMS may be beneficial, although this has not been fully studied. Wu and Liu,21 for example, suggests integrating rTMS with acupuncture; further research is required to confirm and improve these methods.
Despite the great promise of rTMS in terms of reduction in pain scores, the effects on more global QoL domains such as emotional stability and sleep remain unclear. Some studies report improvements in sleep quality and emotional distress,17,18 while others find no significant changes.19 Our results did not show significant differences between the rTMS and the sham groups in the PGIC score at 10 days, 1 month, or 3 months, despite the high level of homogeneity. The subjective character of the test and the limited sample size in the included studies could be the cause of this.
The effectiveness of rTMS is influenced by patient variables like age, the location of pain, and the length of discomfort. Studies on the effects of these factors, however, yield contradictory findings. For example, Ma et al.17 found that patient age and pain duration did not significantly affect rTMS efficacy, whereas other studies suggest these factors are crucial.
rTMS is also well known for its high safety profile, with fewer potential adverse effects, including occasional hearing loss,44 headaches,45 and exceedingly rare cases of seizures,46 provided it is used within defined treatment guidelines.
In general, elderly patients and those with complicated medical conditions who might benefit from forgoing medication for PHN should pay special attention to rTMS. According to our findings, rTMS that targets the M1 may provide long-term pain alleviation for PHN with distinct clinical consequences.
According to this meta-analysis, rTMS at 10 Hz may help alleviate PHN symptoms. However, its long-term effectiveness, impact on QoL and sleep, and durability of effect remain uncertain. Further studies are needed to address these gaps. In the meantime, rTMS may be recommended for elderly patients and those with complex medical conditions as a way to potentially avoid the adverse effects of medications, pending stronger evidence.
In addition, the analysis of cost-effectiveness, especially in relation to standard treatments, is crucial for a thorough assessment of the strengths of rTMS. Treatment expectations relating to rTMS in PHN should also take into consideration these parameters and other individual factors.
While our meta-analysis focused on the efficacy of rTMS in managing pain in PHN, it is important to acknowledge methodological choices made in other related meta-analyses. For instance, a recent study by Dai et al.47 used the SMD to pool outcomes such as pain scores assessed using the VAS.
We would like to point out several key methodological concerns. First, the use of SMD in their analysis, when all studies used the same scale (VAS), may not be the optimal choice. Given that VAS measures pain on a consistent 0–10 or 0–100 point scale across all included studies, the MD or weighted mean difference (WMD) would have been more appropriate.11 Using SMD unnecessarily complicates the interpretation of results by converting them into standard deviation units, which can obscure the real clinical significance. In contrast, MD/WMD would preserve the original units of measurement, making the findings more interpretable and clinically relevant.
Second, Dai et al.47 analyzed post-treatment values rather than changes from baseline, which could lead to misleading findings due to potential baseline differences between the rTMS and sham groups. When baseline values differ between groups, analyzing post-treatment scores without accounting for these initial differences may skew the results. Analyzing the change from baseline is a more accurate approach, as it adjusts for these initial variations and provides a clearer picture of the true effect of the intervention.11 Notably, this decision in their analysis led to highly significant heterogeneity across their results, as reflected by their I2 values. In contrast, our analysis, which focused on changes from baseline, showed non-significant heterogeneity, suggesting a more consistent and reliable estimation of the true treatment effect.
Additionally, Dai et al.47 focused on a limited set of outcomes, namely PGIC, and Short-Form McGill Pain Questionnaire. In contrast, our meta-analysis took a more comprehensive approach, also analyzing sleep quality, QoL, medication regulation, and adverse events. This broader scope provides a more holistic understanding of the impact of rTMS on PHN patients, addressing not only pain but also the broader aspects of patient well-being and safety. Including these additional outcomes offers valuable insights into the full spectrum of rTMS effects, beyond just pain relief, which is crucial for evaluating its overall clinical utility.
Lastly, it is worth noting that the Dai et al.47 study included a trial that could not be located in publicly accessible databases or found on the Internet. Furthermore, this study was not linked or referenced properly in their reference list (see reference number 15 in Dai et al.47), which raises questions regarding its authenticity and the transparency of the data selection process. Ensuring that all included studies are accessible and verifiable is a fundamental aspect of conducting a reliable meta-analysis.
Based on these considerable limitations in previous analyses, we conducted this meta-analysis to provide a more transparent and reliable assessment of rTMS efficacy in PHN. By adopting standard statistical methods, such as focusing on MD for consistent outcome measures, analyzing changes from baseline, and ensuring a comprehensive inclusion of relevant outcomes, we aim to offer more robust and clinically meaningful insights. Our goal is to ensure the findings are interpretable, trustworthy, and readily applicable to clinical practice.
Our current research is limited by several significant factors. Firstly, all our participants were from one ethnic group, the Chinese population.
Therefore, generalizing our findings to other racial groups may not be accurate, as the efficacy of rTMS could vary in different races. More diverse racial samples should be adopted by future rTMS studies, advantages for a better understanding of rTMS benefits.
It is more difficult to interpret our findings because of the observed heterogeneity in the results of the included research, which is caused by variations in the rTMS parameters employed across different investigations, such as the number of stimuli, the time between trains, and the length of sessions. The standardization of such parameters across trials will enhance the consistency and allow for comparisons to be made between different studies, thus facilitating clearer recommendations.
Moreover, the limited size of the conducted studies on rTMS for PHN can affect the strength of our conclusions. Large-scale studies with a wide range of patient demographics are necessary to strengthen the evidence base and draw more reliable conclusions on the efficacy of rTMS in treating PHN.
CONCLUSION
According to the available evidence, rTMS at the stimulus frequency of 10 Hz might contribute to the alleviation of pain sensations in patients diagnosed with PHN. Nonetheless, the time-dependent change in its pain reduction efficacy and the observed lack of significant efficacy in QoL and sleep quality remain questionable and require further attention in future RCT’s. This should be addressed in future research, along with the longer-term impacts of rTMS and its possible advantages in larger patient populations.
Notes
AVAILABILITY OF DATA AND MATERIAL
The data presented in this study are available upon reasonable request from the corresponding author.
AUTHOR CONTRIBUTIONS
Conceptualization: AA, BL, MA, HMM, MEM, MAZ, HS; Data curation: HMM, MEM, MAZ, HS, DEA, AIGS, MSMS, AH, HA; Formal analysis: AA, HS; Investigation: AA, HMM, MEM, MAZ, HS, DEA, AIGS, MSMS; Methodology: AA, HMM, DEA, AIGS, AH; Validation: AA, HMM; Supervision: AMR; Writing–original draft: AA, BL, MA, HA, AMR; Writing–review & editing: AA, BL, DEA, HA, AMR.
CONFLICT OF INTEREST
No potential conflict of interest relevant to this article was reported.
FUNDING STATEMENT
Not applicable.
ACKNOWLEDGMENTS
Not applicable.
SUPPLEMENTARY MATERIAL
Supplementary materials are available from https://doi.org/10.62087/hpr.2024.0032.
The quality assessment of randomized controlled trials using ROB 2
The quality assessment of the case control study according to Newcastle-Ottawa Scale
The leave-one-out analysis to the visual analogue scale.
rTMS, repetitive transcranial magnetic stimulation; SD, standard deviation; IV, inverse variance; CI, confidence interval; df, degree or freedom.
The leave-one-out analysis to the sleep quality.
rTMS, repetitive transcranial magnetic stimulation; SD, standard deviation; IV, inverse variance; Std., standardized; CI, confidence interval; df, degree or freedom.